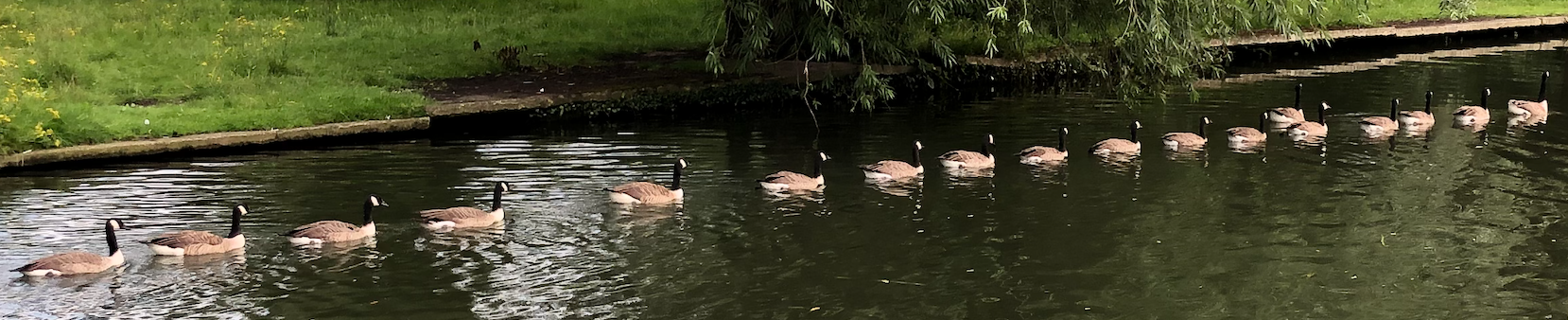
Many-body phenomena describe behaviours of interacting systems, which are typically too complex to calculate by classical computers. Ultracold atoms as quantum simulators provide an alternative approach to investigating these phenomena.
One paradigmatic example is the Mott transition in the Bose-Hubbard model. The interplay between interactions, U and tunnelling, J underlies the quantum phase transition from a superfluid to Mott insulator. This phase transition is usually continuous (or second order), where the system undergoes a smooth change across the transition. We have realised a discontinuous (first order) Mott transition in a shaken lattice. It features hysteresis and metastability, shining light on simulating false vacuum decay in the early universe.
On the other hand, sufficiently strong disorder of the potential, Δ can also localise non-interacting particles, known as Anderson localization. In the presence of both disorder and interactions, a novel Bose glass phase emerges. It is the ground state of interacting disordered systems and a compressible insulator, sitting between superfluid and Mott insulating phases. Interestingly, the competition between interactions and disorder can lead to delocalisation. Studying how systems localise and delocalise is pivotal to applications such as quantum annealing which envisages simulating many NP-complete optimisation problems.
References:
[1] B. Song, S. Dutta, S. Bhave, J.-C. Yu, E. Carter, N. Cooper, U. Schneider, Realizing discontinuous quantum phase transitions in a strongly correlated driven optical lattice, Nature Physics 18, 259 (2022)
[2] J.-C. Yu, S. Bhave, L. Reeve, B. Song†, U. Schneider†, Observing the two-dimensional Bose glass in an optical quasicrystal, Nature 633, 338 (2024)